Linus Pauling: Jack of All Trades, Master of All
As “science keeps marching on,” Pauling has done us a favor by delving into the complex branches that every scientific field has to offer and leaving behind revolutionary findings that the next generation of scientists can now challenge and interpret. Art Request: A drawing of this iconic photo of Pauling teaching back at CalTech
Reading Time: 4 minutes
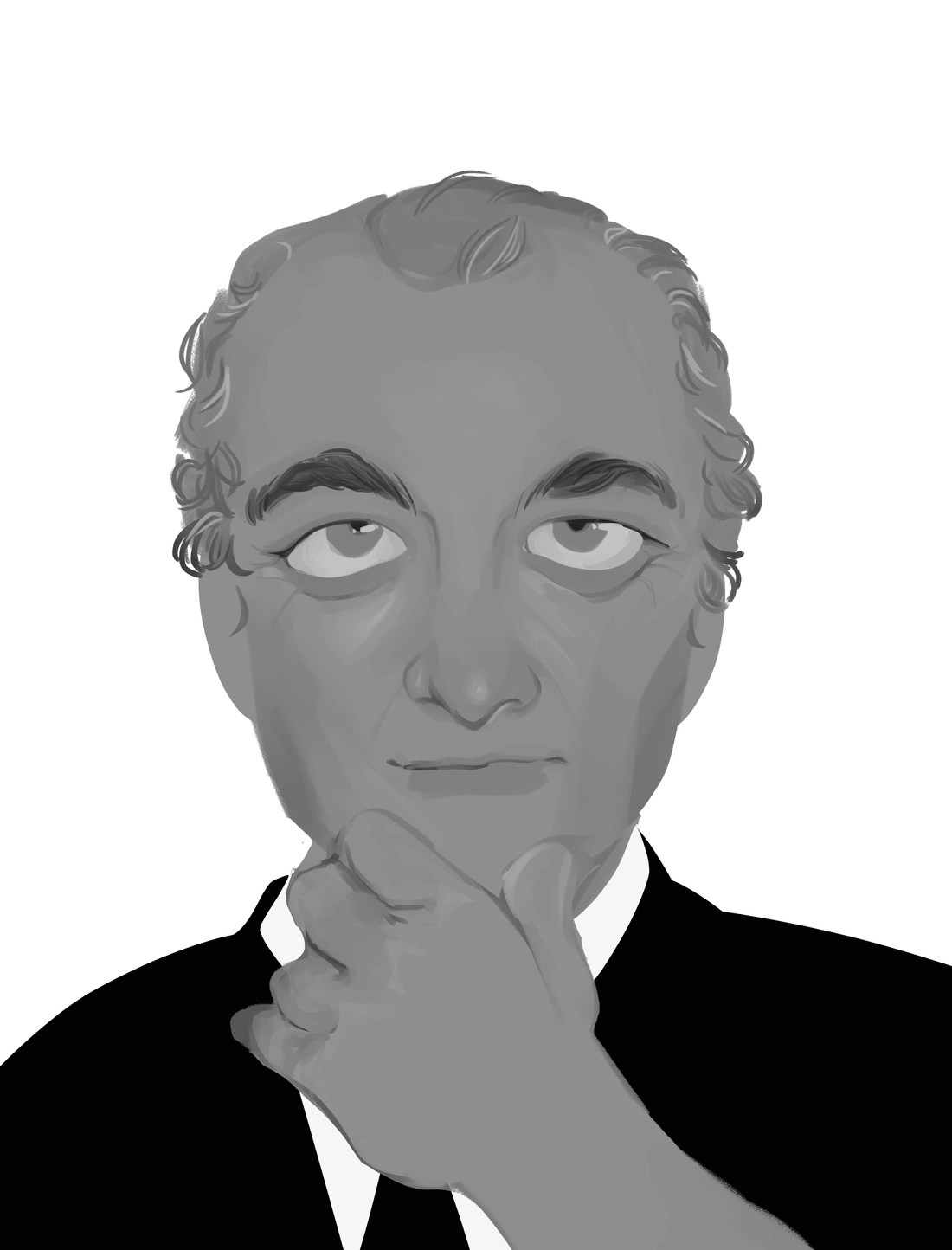
Though many Stuyvesant students consider themselves scientifically oriented individuals, many feel that there is one specific branch of science that is extremely challenging to comprehend. For some it’s biology, for others it’s chemistry, and for myself (and 99 percent of the Stuyvesant population) it’s physics. These “big three” sciences make up the foundations of the scientific world, and mastering the biological, chemical, and physical sciences with appropriate applications allows scientists to apply a conceptual understanding to everything from the largest of organisms to the smallest of particles.
Linus Pauling (1901–1994) was a founding figure of molecular biology and the then relatively new field of quantum chemistry, which uses mathematical models to explore particle motion in chemical reactions. At the young age of 15, Pauling already had enough credits from high school to enter Oregon State University (OSU), where he spent his undergraduate years teaching chemistry with the approval of his professors, who noticed Pauling’s remarkable talent for the subject. During his time at OSU, he was drawn to the fields of mathematical, physical, and theoretical chemistry.
In the 1930s, the scientific community struggled to understand how molecules were oriented. It was pretty clear that atoms and molecules of higher structural order existed, as demonstrated by the discovery of p orbitals defining three-dimensional space by Niels Bohr, but now the looming question was, “How did they look in 3D?”
Facing the challenge, Pauling began to look at the work done by chemists at the California Institute of Technology, where he enrolled shortly after for his doctorate. Scientists such as Gilbert N. Lewis—after whom Lewis dot diagrams are named—were grappling with the concept of valence bond theory. Valence bond theory is founded on the idea that electrons are shared in orbitals, which are areas where an electron is most likely to be found in an atom. Pauling dedicated his life’s work to figuring out what would later be known as The Nature of the Chemical Bond (1939). He used X-ray crystallography, a method that utilizes high-powered radioactive waves to visualize molecules. After shining radiation onto methane, a carbon atom with four hydrogens attached, Pauling realized that it had a tetrahedron (triangular pyramid) structure in 3D space. From this observation, he proposed that orbitals are hybridized, explaining that the direct overlap of electrons allowed chemical bonds—known as sigma (σ) bonds—to form, giving rise to the 3D nature of molecules. Pauling was awarded the Nobel Prize in Chemistry in 1954 for his exceptional research findings.
The extensive nature of discovering 3D molecular structures became more apparent as scientists came across a new problem while dealing with the then-mysterious structure of DNA. Though it is now common knowledge that the DNA molecule is a double-stranded helix, that wasn’t the case 70 years ago. By 1952, Pauling had already used his mathematical applications to find the alpha helices and beta-pleated sheets responsible for the structure of proteins, so the general public had great faith that Pauling would find the structure of DNA. The helix that Pauling proposed had multiple chemical faults, though. For one, he had made a triple helix with too many nucleotides coding for the genetic information in DNA. This made the molecule very unstable and vulnerable to damage. These faults, among others, were building blocks for the eventual finders of the double helix, James Watson and Francis Crick, in 1953.
Despite this inaccuracy in solving the DNA mystery, Pauling’s work in biochemistry holds impressive significance. He used electrophoresis on hemoglobin, a protein that carries oxygen in the blood, to prove that it was present in both its mutant and regular forms in patients diagnosed with sickle cell anemia. Thus, Pauling opened another field of science: molecular genetics, a field that works with how proteins, which are encoded by genes, can lead to potentially life-threatening conditions. Scientists could then diagnose their patients with more confidence and propose certain gene therapies to alleviate symptoms.
Pauling’s work outside the lab was just as influential as his work inside. The rise of nuclear warfare during World War II led to the Manhattan Project, a government-funded project with the world’s leading atomic scientists to make the first nuclear bomb. Pauling challenged President John F. Kennedy, asserting that being in a nuclear arms race would cause biological defects in unborn babies and children who were susceptible to nuclear radiation exposure. Additionally, Pauling argued that it wasn’t ethical to test nuclear bombs on unsuspecting civilians just to demonstrate military dominance, especially following the devastating atomic bombings of Hiroshima and Nagasaki. Under the Emergency Committee of Atomic Scientists, Pauling and other prominent scientists vigorously protested against the American government’s nuclear testing. Kennedy finally complied, signing the Partial Ban Test Treaty into law to suspend all nuclear test detonations. Pauling was then awarded his second Nobel Prize in 1962 for his efforts in nuclear activism.
Pauling’s death in 1994 marked the end of his 93-year career in the big three sciences, laying the foundations of biology, chemistry, and physics that have now become part of our textbooks and high school curriculums. But before you jump to conclusions and blame Pauling for your 65 in AP Chemistry, remember that his contributions to science are now used everywhere in modern medicine and industrialization. Pauling’s ideas about proteins are now used to detect COVID-19 in rapid testing kits; his ideas in particle movement allow engineers to figure out which batteries are the most efficient yet compact sources of energy; and now, geneticists can develop molecular techniques to further explore medical genetics and diagnose immunocompromised patients. And as science keeps marching on, Pauling has done us a favor by delving into the complex branches that every scientific field has to offer, and leaving behind revolutionary findings that the next generation of scientists can now challenge and interpret.