Making Ears Out of Apples
Augmented biology reveals that fruits and vegetables may hold clues to solving some of the world’s largest medical problems.
Reading Time: 5 minutes
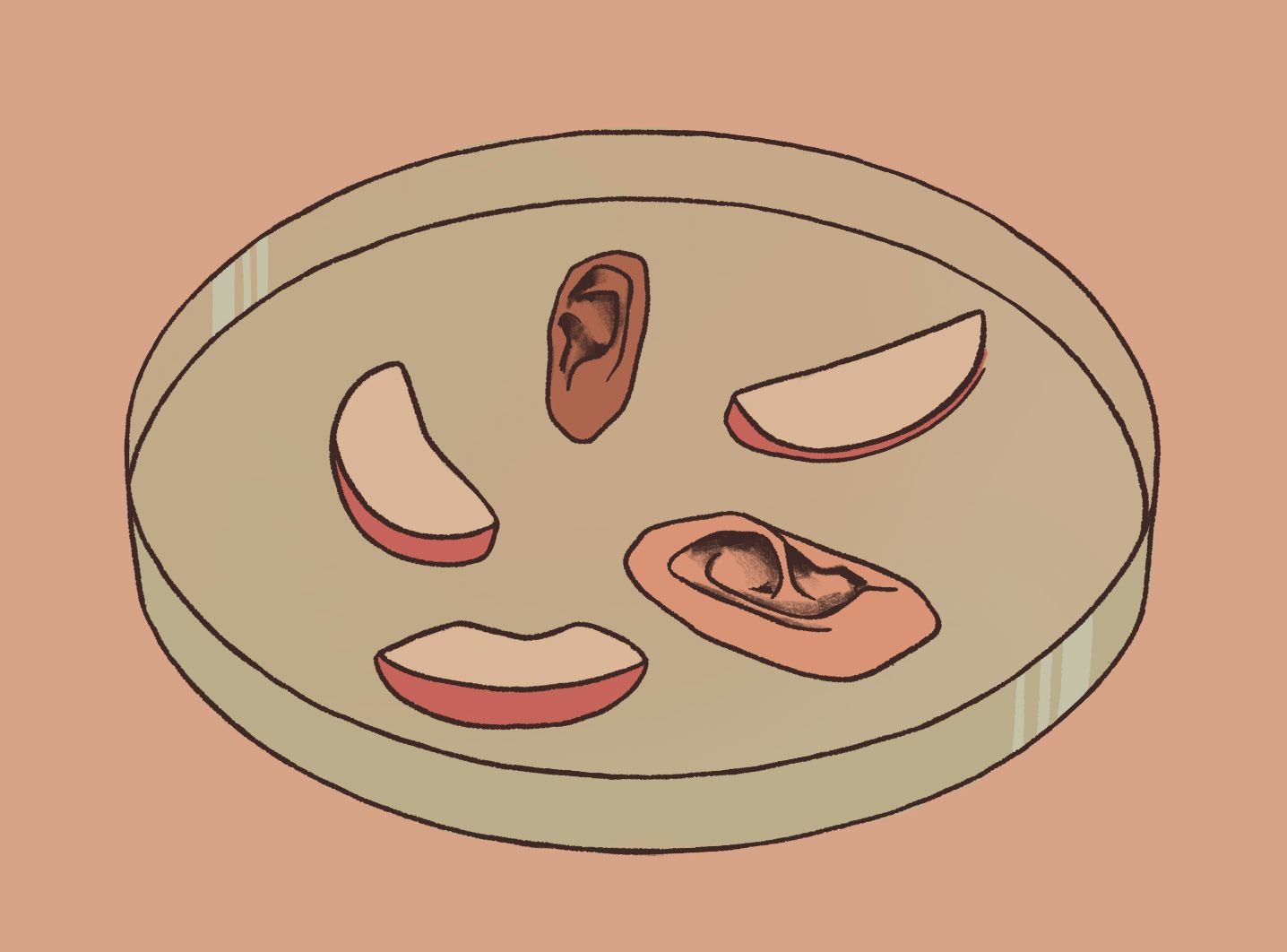
An apple is just an apple. Or is it?
Thanks to Andrew Pelling and his groundbreaking research in the up-and-coming field of augmented biology—the study of creating living, functional biological objects without deliberately modifying genetic material—scientists have begun a more thorough investigation of our classic fruits and veggies. Within their juicy pulp and tough rinds, fresh produce holds something far more potent and appealing to scientists than simply a tasty snack. All fruits and vegetables are rich sources of an organic compound called cellulose. This biological substance is what gives fruits and vegetables both their fiber content and their structural integrity. But this tricky compound cannot be digested by humans, as we lack the enzymes necessary to break the beta-acetal linkages that connect its glucose monomers, nor is it naturally present in the human body.
For this reason, scientists have largely avoided cellulose in generating lab-grown organs, bones, and cartilage. Instead, they opt to use “scaffolds” that are native to the human body, namely cells from donor organs. However, this process is complicated and only simpler organs, like the bladder, have been successfully produced. Bargrat Grigoryan, Ph.D. and co-founder of Volumetric Industries, likens generating an organ to “building a city,” where the organ’s blood vessels and pathways are comparable to a city’s infrastructure: messy yet meticulous. Synthetic alternatives that foster blood vessels from within are no less complex and come with a hefty price tag.
For years, it was commonly believed that these were the only two options for organ generation. Through his research, Pelling changed that. Back in 2016, Pelling and his team at the Pelling Laboratory for Biophysical Manipulation at the University of Ottawa hypothesized that plant cellulose could be used as a scaffold for developing human organs. Pelling reasoned that taking plants and stripping them of their DNA and cells would leave behind only the simplest natural fibers, which could play a key role in reconstructing living tissue. Pelling decided to test his theory on apples.
The team took an average McIntosh apple and removed its cells and DNA. What remained was a translucent, decellularized cellulose scaffold. To assess the biocompatibility of the cellulose biomaterial, Pelling and his two co-researchers, Daniel J. Modulevsky and Charles M. Cuerrier, subcutaneously implanted the samples into mice. The results were astounding.
The mice exhibited a low inflammatory response and a near-complete absence of the manifestations most commonly associated with organ rejection: edema, discomfort, swelling, fatigue, and flu-like symptoms. Four weeks post-implantation, the scaffold featured a vast variety of cell populations, including lymphocytes, monocytes, macrophages, eosinophils, and even foreign body-type multinucleated giant cells (FBGCs), which are commonly found on implanted biomedical devices and are believed to mediate oxidative damage to biomaterial surfaces. The researchers observed extensive vascularization of the implanted tissues eight weeks post-implantation, suggested by the newly formed capillaries and blood cells throughout the cellulose scaffold and in the surrounding areas.
These results led Pelling to believe that the use of plant-derived cellulose biomaterials is a valid approach for generating scaffold implantations. In the study’s conclusion, the researchers acknowledged that “plant-derived materials are cost-effective to produce and extremely straightforward to prepare,” and that their qualities of “clear biocompatibility, an ability to retain their shape while supporting the production of natural extracellular matrix, and most importantly, the promotion of vascularization” make the use of a cellulose scaffold for tissue generation particularly appealing.
The study found that the scaffolds could be easily molded into specific shapes and sizes, which could prove advantageous in generating “personalized” organs and tissues. When Pelling tried injecting human cells into the empty cellulose scaffold, the mammalian cells were observed to proliferate, migrate, and remain visible (with the use of a scanning electron microscope) within the scaffolds. In a separate experiment, Pelling’s wife carved apples into the shape of ears, which the research team then treated to replace the apple cells with human cells. The finished product was an ear composed of real, living human cells built upon a scaffolding of apple cellulose. And from Pelling’s other experiments, researchers now know that this “ear,” or any other biomedically engineered organ formed through the sculpting of plant cellulose, can be safely implanted and incorporated into mammalian tissues.
Pelling’s work introduced an entirely new approach to organ regeneration. The research demonstrated that human cells could thrive on a plant’s fibrous structures. After releasing his findings, though, Pelling did not stop his research at apples.
Pelling’s lab has been expanding upon this knowledge by using asparagus to treat spinal cord injuries. Such injuries can have devastating effects, from pain and numbness to full-scale paralysis, for which there is no accepted treatment plan. To Pelling, the microchanneled vascular bundles present inside the stalks of asparagus bore a striking resemblance to the neuron microchannels of the spinal cord.
While other scientists were using synthetic polymers and animal products in the race for a cure for paralysis, Pelling tried using the same cellulose scaffold that he had retrieved from the McIntosh apple—but this time from asparagus. In their study, the researchers severed the thoracic region of the spinal cord of an animal and then implanted an asparagus scaffold as a bridge between the two detached ends. The asparagus bridge served as a way for neurons to continue transmitting their electrochemical signals despite the damaged spine. Approximately eight weeks after paralysis, the animal recovered from a paraplegic state to walking almost normally. The research revealed that treatments for even the most complex injuries may lie in the astonishing potential of plant tissue. Asparagus may hold the key to battling paralysis’ “incurability”: its cellulose might just be enough to restore feeling and mobility in paralyzed limbs. With the FDA’s designation of the technology as a medical breakthrough device, Pelling’s lab will be translating these discoveries into human clinical trials that will begin in roughly a year.
Augmented biology is proving to be the future of regenerative medicine, now allowing diseases and conditions previously thought untreatable to be cured. Pelling’s work shines light on cellulose’s fascinating ability to direct cell growth in a way that might one day effectively reconstruct human bones, skin, veins, organs, and beyond. What Pelling did with his McIntosh apples is largely referred to as “using trash to make treasure” because plant-based scaffolds represent a low-cost biomaterial that is easily and globally accessible. While many biomedical engineers readily dismissed the proposition of using plant-based scaffolds to generate human tissues because of our inability to chemically break them down, Pelling and his research team revealed that the biological inertness and stability of plant tissue is exactly what makes it so biocompatible with our rapidly regenerating cells. Though the scientific world has become increasingly complex, this research should remind us that even the simplest things can hold the answers to our largest problems.