Organocatalysis: From Metals to Monumental Catalysis
MacMillan’s goal was to find a substitute for reaction mechanisms that involved metals, replacing them with nitrogen compounds to produce iminium and enamine pathways, resulting in durable catalysts with stereochemically high yields and minimal atmospheric waste.
Reading Time: 4 minutes
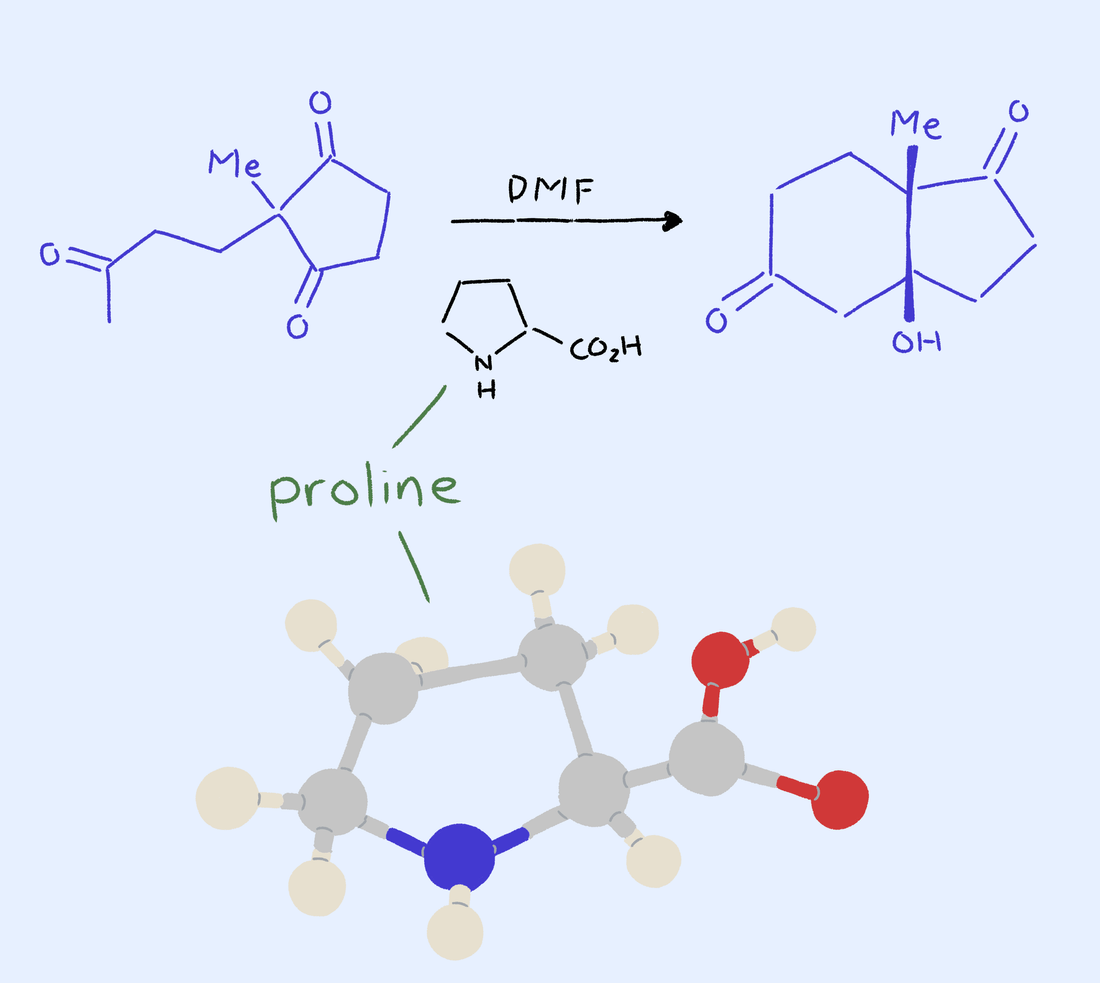
If you have ever taken a good look at your hands, chances are you found nothing wrong with them. Your left hand avidly flips through the pages of your English assignment, while your right hand scrolls through the latest TikTok videos. Your hands, while doing two very different jobs, are also opposites of each other. If you put your hands in front of you and try to layer them on top of each other, it will be impossible to overlap them. This property, known as asymmetry, is one of the key concepts in Nobel Prize-winning chemists Benjamin List and David MacMillan’s contributions to the field of organocatalysis.
To understand the fundamentals of organocatalysis, more specifically referred to as asymmetric organocatalysis, and its use in chemical practice, it is essential to break down the term into its constituents. Catalysis is the act of lowering a reaction’s activation energy, or the amount of energy needed for a reaction to transform reactants into products, improving efficiency and leading to a higher product yield. A catalyst’s role may be to reorient molecules, agitate reactants, or serve as a reactant. The concept of asymmetric reactions, as demonstrated by your hands, can also be applied in organic chemistry to create higher yields of different molecules. In fact, we say that molecules are able to have “handedness”—they are either left-handed (S notation) or right-handed (R notation). These categorizations are fundamental to stereochemistry, which is the study of molecules in 3D space and their effects on chemical reactions. However, depending on whether a molecule is left-handed or right-handed, the properties of the molecule can be completely different, and in some cases, incredibly toxic to the body or other organisms. Toxic molecules are most commonly right-handed. A very famous example is the molecule thalidomide. The left-handed version of the molecule was used as a sedative, while the right-handed version was proven to damage embryos during human pregnancy. Thus, it was an extremely crucial task for chemists to confirm that their catalysts had a higher yield of the left-handed product than the right-handed product.
“Organo-” refers to the usage of organic molecules rather than enzymes or metals, as catalysts. In their research, List and MacMillan identified two problems with the two existing types of catalysis they were investigating. Enzymes are incredibly specific to their substrate complexes, and under certain environmental stressors, such as heat and pH changes, protein structures denature, preventing molecules from binding to the enzymes to be activated. Metal catalysts are often preferred because of their ability to exist in different oxidation states, which refers to atoms in different ionic forms. The same goes for many other transition metals, including zinc, copper, and platinum. However, the release of metal cations, or molecules with a positive charge, into the atmosphere allows for the formation of dangerous heavy metals such as palladium, which are toxic to humans. MacMillan’s goal was to phase out metal catalysts by using compounds composed of carbon, nitrogen, and hydrogen to create the same products that metal catalysts would have. All of this would be done with little to no pollution into the atmosphere—a significant step toward sustainable scientific research.
MacMillan and his team worked to make a 90 percent left-handed yield using the Diels-Alder reaction mechanism as a basis. Using molecular engineering, MacMillan’s team managed to alter the shape of the first-generation catalyst they had originally designed by adding two more methyl groups, resulting in 95 to 99 percent desirable yields. This allowed for other existing reaction mechanisms, in which catalysis could be performed with organic molecules as substitutes for metals in hundreds of different reactions. Scientists would no longer have to rely on the costly and inadequate supply of metal catalysts.
The applications of organocatalysis are still fairly new, but many chemists have already started to utilize this process in environmental chemistry to break down plastics, including polyethylene terephthalate (PET). For over a decade, environmental scientists have been exploring ways to efficiently reduce plastic waste in aqueous bodies, as well as maintain the quality of the plastic constant when it is reused. Recent studies in the Green Chemistry journal found that scientists used a catalyst known as tetramethylammonium methyl carboxnate to break down PET into its monomer, dimethyl terephthalate, while simultaneously preserving the PET polymer’s traits. This proves that the chemical recycling of plastic can be achieved sustainably through organocatalysis without producing mass plastic waste in oceans.
Perhaps the most exciting news is for scientists in the pharmaceutical and biochemical industries; organocatalysis can be used to assist in biochemical reactions, producing molecules that trigger cell signaling pathways within living organisms. As of now, organocatalysis has used methyl nitrite as a catalyst to produce drugs that can be used to treat migraines caused by hypocalcemia, calcium deficiencyin the blood. With further testing, scientists hope to be able to prescribe this molecular drug to prevent future hospitalizations that occur from active transport malfunction.
The future of organocatalysis is one that scientists do not have to worry about cost, hazardous waste, or accessibility. It comes down to what people can make using the simple nitrogenous catalyst pathways proposed by List and MacMillan. The possibilities—perfumes, medicine, furniture manufacturing—are quite endless, as endless as a chemist’s imagination.