Printing Life: 3D Custom Hearts
Revolutionary aortic stenosis treatments use printed 3D custom hearts.
Reading Time: 4 minutes
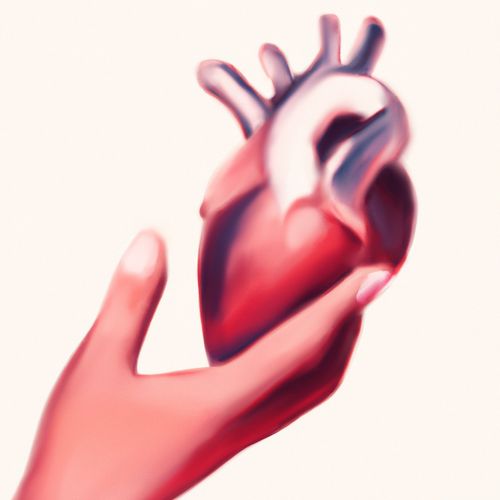
Thump thump thump. One hundred thousand times a day, a fist-sized organ beats as we walk, read, write, talk, and sleep. Protected by our skin, strong muscles, delicate tissue, and ribcage is one of the most vital organs in the human body: the heart. The circulatory system enables oxygen and nutrient-rich blood to be transported throughout the body, which is key in maintaining organ homeostasis. The heart is the center of the circulatory system, connecting a network of arteries, veins, and capillaries—all of the delicate equipment needed for survival.
Each heart is unique; every heart is a different size depending on body shape, body mass, and genetic factors. However, most healthy hearts have the same fundamental elements: four hollow chambers surrounded by muscles and heart tissue. The upper two chambers, the atria, are where blood flows from the body and lungs to the heart, while the lower two chambers, the ventricles, are where blood is pumped from the heart to the lungs and the rest of the body.
The heart functions using the pulmonary (lungs) and systemic (full body) circuits, each of which has distinct functions. The pulmonary circuit carries deoxygenated blood from the heart, cycling it to the lungs and returning it back to the heart. On the other hand, the systemic circuit carries oxygenated blood, cycling it through the rest of the body and returning it to the heart. These processes are supported by veins and arteries, which bring blood to and from the heart. In order to control blood flow, functional heart valves are crucial. Heart valves are gates that allow blood to flow in and out of the heart and prevent it from flowing backward by opening and shutting their flaps.
Aortic stenosis is a heart valve disease in which the left ventricles and aorta do not fully open, resulting in reduced blood flow. When this occurs, the heart must work harder to pump blood, causing the left ventricle to thicken and enlarge from overexertion. Eventually, this overexertion weakens the cardiac muscles and can cause heart failure. Medications such as ACE inhibitors and beta-blockers can resolve internal issues contributing to valve failure, like blood clotting, but they have minimal effects on severe versions of the disease. The only known option to treat extreme cases of aortic stenosis is heart valve surgery.
In heart valve replacement surgery, a surgeon replaces damaged or diseased heart valves by implanting synthetic valves designed to widen the aorta. However, there is a high probability of heart damage from valve infections, which result from foreign germs attacking the heart’s internal structures. Every heart also has unique valve sizes, so an inaccurate measurement of the synthetic valves can lead to blood regurgitation within the pulmonary or systemic systems. These problems can accompany more serious risks, including nerve damage, organ damage, and the rupture of the heart wall.
For years, researchers have looked to combat aortic stenosis without the complications that can arise from valve replacement surgery. The goal is to use a custom-printed heart to compare implants of different sizes and find the best fit and flow for each heart. This development would allow clinicians to determine the ideal valve size for implantation in cases of severe aortic stenosis, lowering the risk of complications.
Dr. Ellen Roche and her team at the Massachusetts Institute of Technology began the development of the hybrid heart in January 2020. The hybrid heart is a simple replica made of synthetic muscles and a blend of two polymers—a stretchable cyclic copolymer elastomer and a much stiffer thermoplastic polyethylene—containing small inflatable cylinders to mimic the contractions of a beating heart. Eventually, the researchers expanded their approach to developing sleeves and heart replicas for individual patients, eliminating the risk of incorrect valve sizes.
To create these hearts, the team first used medical scans of 15 patients diagnosed with aortic stenosis, converting the scans into a 3D model of a typical aortic stenosis patient's left ventricle and aorta. Then, they fed the model into a 3D printer to generate a soft, unique shell of the ventricle and vessels. The 3D ventricles and vessels were composed of polymer ink, a mix of natural and synthetic substances used for 3D printing that are squeezable and stretchable. The researchers also wrapped fabricated sleeves around the 3D hearts and connected them to a small air-pumping system. Then, these sleeves were customized to contract and constrict the 3D models like authentic hearts do.
Furthermore, the researchers mimicked patient surgeries to see whether the printed heart and vessels would respond similarly to an authentic heart. When the results came back identical to an authentic heart, the researchers used valve implantation patient models to implant the valves in their printed heart. Once again, they achieved similar results as valve implantation patients. In other words, their experiment was a success.
Currently, the model heart is used to recreate an accurate rate of heart cramping pressure and flow for individual patients. Using these patient-specific models, researchers can develop and identify ideal treatments for those with even more complicated cardiac diseases. However, 3D custom printing is also extremely expensive, with specialized bioprinters costing upwards of $100,000. These high prices are exclusionary. Hopefully in the future, 3D custom hearts will become more affordable, but until then, this innovation can enable scientists to save more lives and drive medicine to unbelievable new heights.