What’s Up With QED?
Quantum electrodynamics (QED) is an elusive topic discussed in modern physics. Learn more about the history of QED and the behavior of subatomic particles and electromagnetic radiation!
Reading Time: 5 minutes
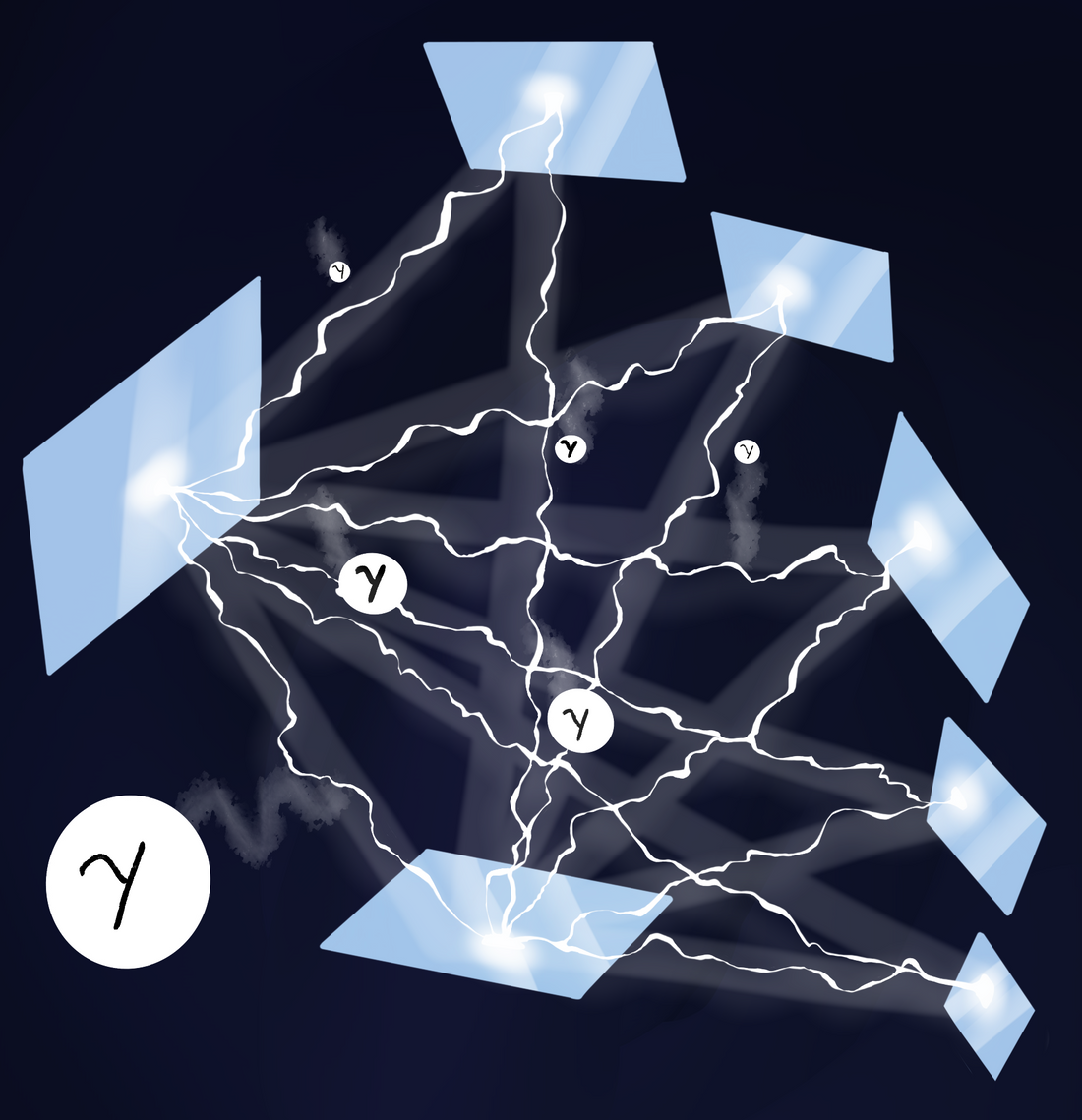
In a horror movie, when there is a mysterious sound heard, the main character either runs for their life or approaches it out of curiosity. This is similar to the interactions between subatomic particles whose fundamental behavior stems from their attractions and repulsions. Modern physics today often focuses on the microscopic realm involving quantum electrodynamics (QED), a combination of quantum mechanics (QM) and special relativity. QM incorporates the calculation of quantum properties and behavior of nature in the subatomic world, which is unpredictable and wild. Later, QM was connected to special relativity—how the speed of an object affects mass, time, and space—with the studies of British physicist Paul Dirac and his “wave equation” fundamentally beginning QED, and later Feynman, with the idea that charged particles interact by emitting protons in the form of gamma rays. Fundamentally, QED describes the unique interactions (or calls) between light and subatomic particles, such as electrons, photons, or muons (μ), which create the world we live in today.
The study of QED stemmed from the photoelectric effect proposed by Albert Einstein in 1905 and Thomas Young’s famous double-slit experiment in 1801. The photoelectric effect describes how electrically charged particles, like electrons or ions, are released when electromagnetic radiation strikes a surface. Electromagnetic radiation is energy, namely photons, consisting of magnetic and electric fields flowing at the speed of light. The electromagnetic radiation released can range from high-frequency gamma rays to low-frequency radio waves. The photoelectric effect gave way to the concept of wave-particle duality, the idea that everything exhibits both particle and wave behavior. The ejection of electrons demonstrated particle-like behavior while the vibration of electrons when struck by electromagnetic radiation demonstrated wave-like behavior.
Wave-particle duality had also been proven by the double-slit experiment. Thomas Young set up a thick wall with two thin parallel slits. Behind the screen was a detector for recording photons. Young shone a light at the wall which passed through the slits. If photons were just particles, then the detector would detect photons that come directly in front of the slits. Instead, an interference pattern had formed, which consisted of alternating bands of photons and no photons. This demonstrated wave behavior because areas where no photons were recorded resulted from destructive interference—the peak (highest point on a wave) and trough (lowest point on a wave) of two different waves cancel out. Meanwhile, the areas where photons were recorded resulted from constructive interference—two peaks or tough troughs combined to form a larger peak or trough. The photons are also particles since each individual photon is recorded by the detector (discernable dots were observed). However, the general public might still wonder why wave-particle duality of everyday objects isn't obvious. This duality is more apparent within the subatomic scale because an object with larger mass has a shorter wavelength (distance between identical points on a wave), making the object less wave-like. As a result of both Einstein's photoelectric effect and Young’s double-slit experiment, interactions between matter and light cannot be solely explained by classical physics which treated all matter and light as particles.
However, physicists faced the issue of the clash between general relativity and quantum mechanics. General relativity, also developed by Einstein, is the theory that matter bends spacetime (space and time are interconnected) like balls curving a rubber sheet that they are placed on. The amount of warping is directly related to the object’s mass. In general relativity, events in the universe are continuous and agree with causality—in other words, each event in the past corresponds to an event in the future. However, in quantum mechanics, events are probabilistic which contradicts the deterministic classical physics. This nondeterministic behavior is attributed to Heisenberg’s Uncertainty Principle. Physicist Werner Heisenberg asserted that it is impossible to know, with absolute certainty, both an object’s position and momentum. This uncertainty exists due to the wave behavior of matter. Since waves can touch multiple points in space simultaneously, one cannot definitely determine an object’s position in space. As a result, future events are only probabilistic outcomes. To resolve this uncertainty, Paul Dirac began QED by formulating the “wave equation,” or more commonly known as the Dirac Equation. The Dirac Equation successfully described the nature of quantum particles like electrons, making them easier to research and comprehend. Additionally, it predicted the existence of antimatter, the equivalent of normal matter but with the opposite electric charge. Examples of antimatter include positrons and antiprotons.
Furthering QED, Richard P. Feynman—one of the greatest contributors to QEM—developed many theories and “simplified” the visualization of QEM greatly with Feynman diagrams. Feynman diagrams, which represent complex mathematical formulas, make QEM easier to understand for beginners and physicists alike. These diagrams are diverse and have thousands of possible combinations and interactions between electrons, positrons, and other subatomic particles or antimatter. When an electron emits a virtual proton in a gamma wave, the receiving electron is either repelled or attracted to the messenger electron.
However, this is only a snippet of particles’ true nature. For example, interactions don’t always need to only happen between two electrons; they can also occur between an electron and a positron—which annihilate each other. Sometimes, loops can be created, which is when virtual particles—particles that only live for a short period of time—and antiparticles annihilate each other. Using Feynman diagrams, visualizations of all these interactions become simplified, and assist in the understanding of complex QED formulas.
In 1949, Feynman and John Archibald Wheeler collaborated to create the Absorber Theory, eliminating possible infinite values in QED calculations with four essential principles. Firstly, when a particle is speeding up with no other particles surrounding it, it will not release light or generate an electromagnetic field. Secondly, particles receive “calls from other particles” and are either repulsed or attracted by those calls. Thirdly, if a particle is emitted, there must always be another particle to absorb it. Finally, the future and the present must be accounted for to predict the particle’s movement. This revised QED theory pushed the walls of QED and made it more accurate and precise, helping QED become one of the most known subsections of the quantum world.
Although QED seems remote and only applicable in the subatomic world, the concepts of QED can be taken advantage of in the macroscopic world. Surprisingly, QED finds itself in transistors, which are found in semiconductors, and is used to map out electron movement. This is because transistors are very small (about 20 nanometers), so quantum physics plays a crucial role in its function. Additionally, electron microscopes use QED because one must understand the interaction between the particles the microscope sends out and the particles it is trying to observe. Other applications include laser absorption and emissions, solar cells, and photodetectors to name a few.
Overall, QED is a complex branch of modern physics that explores the interactions between subatomic particles and electromagnetic radiation. From discovering wave-particle duality to Absorber Theory, QED has been a field that gradually emerged and evolved over time. While most people aren’t familiar with QED as it is a difficult concept to grasp, QED plays a big role in our daily lives. As more discoveries in QED continue to be made, QED can be used for further beneficial applications.